Sequencing technology shows proteins can interact differently in the same plant, allowing more variety improvement opportunities
New genetic research on corn has found that the plant is much more diverse than scientists first believed, or even hoped, when they decoded its genome in 2009.
The discovery promises to open up all kinds of opportunities.
Corn is the No. 1 cereal crop grown in the world and accounts for two-thirds of world food production along with rice and wheat.
In Canada, it is the third largest crop behind wheat and canola and is grown largely in Ontario and Quebec.
The crop’s productivity has been enhanced by improved farming practices and advancements in biotechnology and breeding. It is used for human consumption, livestock feed and as an industrial commodity.
Read Also
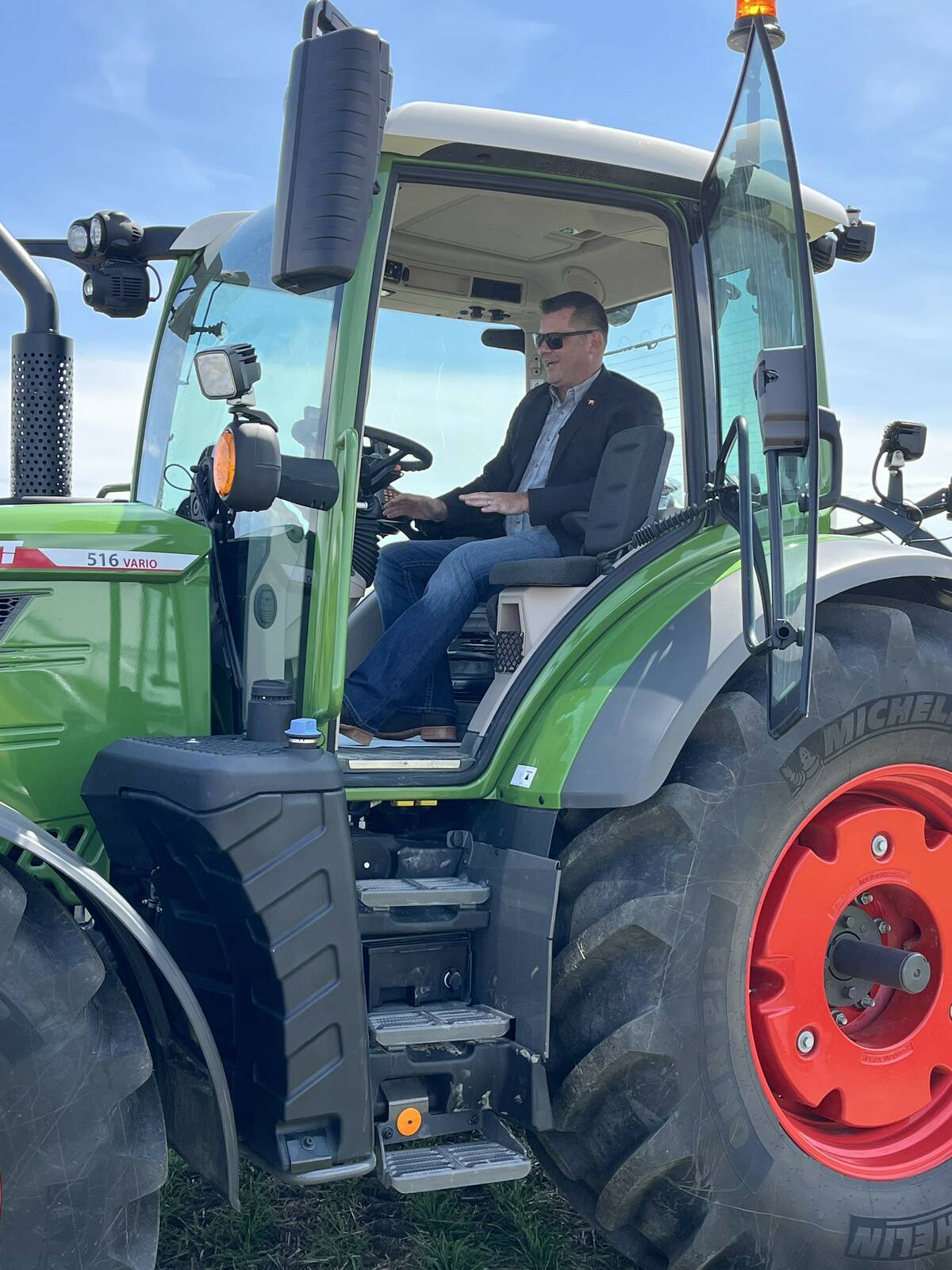
Farming Smarter receives financial boost from Alberta government for potato research
Farming Smarter near Lethbridge got a boost to its research equipment, thanks to the Alberta government’s increase in funding for research associations.
A greater understanding of the plant’s potential at the molecular level is now helping scientists push the boundaries on corn’s many applications.
“Our new research establishes the amazing diversity of maize (corn), even beyond what we already knew was there,” says Doreen Ware of the U.S. Department of Agriculture and the Cold Spring Harbor Laboratory in New York.
Ware was part of a large multinational team that in 2009 assembled the first-ever sequence of corn’s 30,000 genes based on a single variety called B73.
Each corn plant is potentially different from the next, even within the same variety, because genes are expressed differently depending on conditions specific to the individual plant — both internally and in its immediate environment such as soil quality, moisture, available nutrients and light.
Each gene contains a set of instructions for making the molecules that the plant needs to survive. The organism doesn’t use the gene itself; rather, it uses a product of the gene known as RNA or protein.
Gene expression is the process by which the gene’s information becomes that product. Since the gene never leaves the cell’s nucleus, the process starts by the gene making a copy of itself, called the messenger RNA. This RNA conveys the DNA codes to the sites for protein synthesis.
“The genome is like the underlying framework for the building blocks (protein) to come out,” said Ware.
“In the first draft of the genome we studied in 2009, we had usually one protein coming out (expressed). This current research shows that there could be several different proteins or building blocks coming out, depending on which cell or the age of the plant. Think about Legos and the long and the short pieces, each fitting differently but all working together. We know there are many different pieces of protein that come together and interact. From this work, we now understand we have a much higher combination. For each location where you might have proteins coming out, where and when each comes out will impact who its ‘partners’ are and the functionality in the long term, making it more complex. Look at the opportunities that you could have.”
The discovery of this extraordinary protein diversity in corn was made possible by a new generation of highly accurate “long-read” sequencing technology, which allows scientists to read many more molecules in their entirety at once. The technology was provided through a research partnership with CSHL and PacBio, a sequencing company.
The technology identified previously unknown genes, although fewer in number.
However, it also showed that many more RNA messages were generated when genes were expressed in proteins of different shapes and functions.
The researchers read and analyzed 111,151 RNA transcripts from genes being expressed in six different corn tissues. Fifty-seven percent of those RNA messages had never been seen and therefore never sequenced.
“These were the messages that told us that our efforts to annotate and characterize the 2009 maize reference genome have been far from complete,” said Bo Wang, a postdoctoral investigator in Ware’s lab and first author of the paper reporting the new research.
Alternative RNA splicing occurs in nearly all forms of multicellular life, including humans. The current research suggests how extensively alternative splicing, and therefore alternative developmental possibilities, are available to each corn plant.
The technology is available for all crop plants, although Ware said the wheat’s genome is so large at 17 billion base pairs that it is difficult to obtain a robust referenced genome for each of the different varieties.
“We may be able to look at the functional part of the genome, two wheat varieties, and how they perform more directly using this approach,” Wang said.
“It may turn out that the differences that may impact a yield outcome could be associated with expression differences at different time and space in the plant, and we could assay this more directly than we could before. The technology is allowing us to address more crops in the species. It will accelerate improvement for the germplasm and longer-term sustainability for agriculture.”
Ware said some proteins may eventually become markers that suggest recommendations for management practices, disease or stress conditions such as reduced nitrogen or drought. However, the technology is not there yet.
“We can find out more about how a plant can adapt to a changing future by monitoring these proteins,” she said.
“With climate change in Canada, all of you are going to be in much better shape with regard to drought. Waterlogging at an early stage is devastating as well, but drought is going to be a major concern moving forward in the future years. This work allows us to unravel the different products the genome can make and gives us insight into how each of the different maize plants may function under different conditions. There’s still so much to find out.”
The research was published in the journal Nature Communications.